Regenerative Medicine Strategies for Treating Neurogenic Bladder
Article information
Abstract
Neurogenic bladder is a general term encompassing various neurologic dysfunctions of the bladder and the external urethral sphincter. These can be caused by damage or disease. Therapeutic management options can be conservative, minimally invasive, or surgical. The current standard for surgical management is bladder augmentation using intestinal segments. However, because intestinal tissue possesses different functional characteristics than bladder tissue, numerous complications can ensue, including excess mucus production, urinary stone formation, and malignancy. As a result, investigators have sought after alternative solutions. Tissue engineering is a scientific field that uses combinations of cells and biomaterials to encourage regeneration of new, healthy tissue and offers an alternative approach for the replacement of lost or deficient organs, including the bladder. Promising results using tissue-engineered bladder have already been obtained in children with neurogenic bladder caused by myelomeningocele. Human clinical trials, governed by the Food and Drug Administration, are ongoing in the United States in both children and adults to further evaluate the safety and efficacy of this technology. This review will introduce the principles of tissue engineering and discuss how it can be used to treat refractory cases of neurogenic bladder.
INTRODUCTION
Many disorders of the genitourinary tract can become severe enough to eventually require reconstruction. These include congenital disorders such as myelomenigocele or bladder exstrophy, bladder cancer, trauma, and chronic inflammation resulting from interstitial cystitis or other conditions. In addition to structural damage, insult to the nerves that innervate the bladder can also lead to bladder dysfunction that is severe enough to warrant surgical intervention and eventual reconstruction of the lower urinary tract. The term "neurogenic bladder" is used to describe these alterations in bladder function that are provoked by neurologic dysfunction that results from disease or injury, and this condition often significantly increases the morbidity of the underlying condition [1]. For example, pathologies that occur at or above the brain stem may cause neurogenic bladder (e.g., cerebrovascular disease, cerebral palsy, Parkinson's disease, traumatic brain injuries, and brain tumors). In addition, pathologies involving the spinal cord (e.g., sacral agenesis, tethered spinal cord, traumatic cord injuries, multiple sclerosis, and transverse myelitis) may also lead to neurogenic bladder dysfunction [2,3]. The most common cause of neurogenic bladder in children is spina bifida, which affects one in one thousand newborns [4].
Bladder dysfunction associated with neurogenic bladder may manifest in different ways [5]. Symptoms of neurogenic bladder range from severe urinary retention to incontinence, and result from detrusor underactivity to overactivity, depending on the site of neurologic insult. The urinary sphincter also may be affected, resulting in sphincter underactivity or overactivity and loss of coordination with bladder function [6,7]. Urodynamic evaluation is important to define aspects of bladder and sphincter function such as bladder overactivity or underactivity associated with either sphincter synergy or dyssynergy [2]. The most serious situations occur with high intravesical or leaking pressures (above 40 cmH2O) and detrusor-sphincter dyssynergy because they represent a potential risk for upper tract deterioration. Children with myelomeningocele and neuropathic bladder, if not managed properly, face a ≥50% risk for upper urinary tract deterioration [8,9]. In these cases, conventional management often consists of pharmacotherapy and frequent catheterization to reduce intravesical pressure and maintain renal function, which can lead to mechanical damage to the urethra and frequent urinary tract infections. In addition, both retention and incontinence considerably decrease the quality of life of the patient [7]. Controlling, or at least minimizing, urinary incontinence and urinary tract infections facilitate the patients' social inclusion and rehabilitation [5,10]. Treatment for neurogenic bladder can also be surgical; some of these options are minimally invasive (e.g., Botox injections and neurostimulator implants) and some are more invasive and reconstructive in nature. In the latter case the diseased bladder is augmented with a bladder dome built from intestinal tissue. This reconstructive surgery is frequently performed in infants to prevent or delay the need for dialysis or kidney transplantation. However, use of intestinal segments in the urinary tract carries significant risks, such as excessive mucus production, urolithiasis, metabolic disturbances, and malignancy [2,10,11].
Because of the problems encountered with the use of gastrointestinal segments, numerous investigators have attempted alternative reconstructive procedures. These have included autoaugmentation [12,13] and ureterocystoplasty [14-16], but neither of these techniques has been entirely successful. Thus, novel methods for bladder reconstruction based on the relatively new field of regenerative medicine are being explored. The term "regenerative medicine" was coined in 1999 by William Haseltine, who was then the Scientific Founder and Chief Executive Officer of Human Genome Sciences [17]. The field of regenerative medicine combines the areas of stem cell biology, cloning, cell transplantation, and tissue engineering with the goal of regenerating living tissues and organs. This review focuses specifically on the novel tissue engineering-based regenerative strategies that are being applied to bladder reconstruction, and discusses how they can be used to treat the neurogenic bladder.
THE BASICS OF TISSUE ENGINEERING
Tissue engineering employs aspects of cell biology and transplantation, materials science, and biomedical engineering to develop biological substitutes that can restore and maintain the normal function of damaged tissues and organs. These include injection of functional cells into a nonfunctional site to stimulate regeneration and the use of biocompatible materials to create new tissues and organs. The introduction of cells is designed to stimulate regeneration, promote vascularization, and/or supplement the production of hormones and growth factors. Biomaterials, which include both natural and synthetic matrices that are commonly called scaffolds, are important tools in regenerative medicine. In addition to guiding the direction of new tissue growth and providing the proper spatial environment to restore tissue structure and function, biomaterials may introduce bioactive factors [18,19] or may attract cells and factors from the body following implantation [20,21]. If a biomaterial is implanted without cells, the objective is to encourage the body's natural ability to repair itself.
Biomaterials Used in Genitourinary Tissue Construction
Synthetic materials have been used widely for urologic reconstruction. Silicone prostheses have been used for the treatment of urinary incontinence with the artificial urinary sphincter and detachable balloon system, for treatment of vesicoureteral reflux with silicone microparticles, and for impotence with penile prostheses [22-24]. There has also been a major effort directed toward the construction of artificial bladders made with silicone. In some disease states, such as urinary incontinence or vesicoureteral reflux, artificial agents (Teflon paste, glass microparticles) have been used as injectable bulking substances; however, these substances are not entirely biocompatible.
For regenerative medicine purposes, there are clear advantages to using degradable, biocompatible materials that possess similar physical properties to native tissue and promote cellular interaction and tissue development [20,21,25]. Three different classes of biomaterials are used in regenerative medicine: naturally derived materials (e.g., collagen, keratin, and alginate), acellular tissue matrices (e.g., decellularized submucosa from bladder or small-intestinal tissue), and synthetic polymers (e.g., polyglycolic acid [PGA], polyactic acid, and polylactic-coglycolic acid). Naturally-derived materials and decellularized tissue matrices are considered to have biological properties that better mimic native tissue or organ extracellular matrix (and are thought to elicit fewer immune responses), but these are limited in supply and have compositions that are difficult to control or even characterize. On the other hand, synthetic scaffolds can be produced on a large scale with controlled properties of strength, microstructure and degradation rate.
Cells for Urogenital Tissue Engineering Applications
Often, when cells are used for tissue engineering, donor tissue is removed and dissociated into individual cells, which are then either implanted directly back into the host or expanded in culture, attached to a support matrix, and then implanted. Ideally, this approach allows lost tissue function to be restored or replaced in toto with limited complications [26-31]. The donor tissue used for this purpose can be heterologous, allogeneic, or autologous.
Autologous cells are the ideal choice, as their use circumvents many of the inflammatory and rejection issues associated with a non-self donor. In the past, one of the limitations of applying cell-based regenerative medicine techniques to organ replacement was the inherent difficulty of growing certain human cell types in large quantities. However, the discovery of native targeted progenitor cells in virtually every organ of the body has led to improved culture techniques that have overcome this problem for a number of cell types. Native targeted progenitor cells are tissue specific unipotent cells derived from most organs. The main advantage of native targeted progenitor cells in terms of regenerative medicine is that they are already programmed to become the cell type needed, and no in vitro differentiation steps are required for their use in the organ of origin. These cells can be obtained from the specific organ to be regenerated, expanded, and used in the same patient without rejection, in an autologous manner [26-29,32-42].
By noting the location of the progenitor cells, as well as by exploring the conditions that promote differentiation and/or self-renewal, it has been possible to overcome some of the obstacles that limit cell expansion in vitro. As an example relevant to the treatment of neurogenic bladder and other urogenital disorders, urothelial cell culture has been vastly improved in this way. In the past, urothelial cells could be grown in the laboratory setting, but with only very limited success. It was believed that urothelial cells had a natural senescence program that was hard to overcome. However, several protocols have been developed over the last two decades that have improved urothelial growth and expansion [34,43-45]. A system of urothelial cell harvesting was developed that does not use any enzymes or serum and has a large expansion potential. Using these methods of cell culture, it is possible to expand a urothelial strain from a single specimen that initially covers a surface area of 1 cm2 to one covering a surface area of 4,202 m2 (the equivalent area of one football field) within 8 weeks [34].
Now, bladder, ureter, and renal pelvic cells can all be harvested, cultured, and expanded in a similar fashion. Importantly, normal human bladder epithelial and muscle cells can be efficiently harvested from surgical material, extensively expanded in culture, and their differentiation characteristics, growth requirements, and other biologic properties can be studied [34,36, 37,44-52]. However, a major concern with this approach has been that, in cases where cells must be expanded from a diseased organ, there may no longer be enough normal cells present in that organ to begin the process. Recent research suggests that this may not be correct. One important study has shown that cultured neuropathic bladder smooth muscle cells possess and maintain different characteristics than normal smooth muscle cells in vitro, as demonstrated by growth assays, contractility and adherence tests in vitro [53]. Despite these differences, however, when neuropathic smooth muscle cells were cultured in vitro, and then seeded onto matrices and implanted in vivo, the tissue engineered constructs showed the same properties as the constructs engineered with normal cells [54]. It is now known that genetically normal progenitor cells, which are the reservoirs for new cell formation, are present even in diseased tissue. These normal progenitors are programmed to give rise to normal tissue, regardless of whether they reside in a normal or diseased environment. Therefore, the stem cell niche and its role in normal tissue regeneration remains a fertile area of ongoing investigation. However, in some instances, primary autologous human cells cannot be expanded from a particular organ, such as the pancreas, or there is not enough normal tissue remaining in the diseased organ to use for the procedures described above. In these situations, pluripotent human stem cells are envisioned to be an ideal source of cells, as they can differentiate into nearly any replacement tissue in the body. For example,embryonic stem cells exhibit the ability to proliferate in an undifferentiated, but still pluripotent state (self-renewal), as well as the ability to differentiate into a large number of specialized cell types, including genitourinary cells [55]. However, there are several problems associated with the use of embryonic stem cells in tissue engineering. Importantly, these cells tend to form teratomas when implanted in vivo, and this risk of tumor formation limits their clinical application. In addition, many uses of these cells are currently banned in a number of countries due to the ethical dilemmas that are associated with the manipulation of human embryos in culture.
As a result, scientists have turned to other types of pluripotent cells for use in tissue engineering. These other cell types include cells derived from the adult, such as hematopoietic stem cells and mesenchymal stem cells [56-59], and cells derived from fetal sources, such as amniotic-fluid and placental-derived stem cells, which were discovered in 2007 [60]. Each of these cell types has been differentiated into epithelial and smooth muscle phenotypes, and thus they may have a role in genitourinary tissue engineering.
Somatic cell nuclear transfer, or cloning, can serve as another source of pluripotent, "stem" cells that could possibly be used for regenerative medicine therapies. In this procedure, a nucleus from a somatic cell from a donor is placed into an ovum, and the resulting cell is induced to divide using chemicals or electricity. This process produces a blastocyst that is genetically identical to the donor and can be grown in culture to produce embryonic stem cell lines. These autologous stem cells have the potential to become almost any type of cell in the adult body, and thus would be useful in tissue and organ replacement applications [61].
Recently, exciting reports of the successful transformation of adult cells into pluripotent stem cells through a type of genetic "reprogramming" have been published. Reprogramming is a technique that involves de-differentiation of adult somatic cells to produce patient-specific pluripotent stem cells, without the use of embryos. Cells generated by reprogramming would be genetically identical to the somatic cells (and thus, the patient who donated these cells) and would not be rejected. Takahashi and Yamanaka [62] were the first to discover that mouse fibroblasts could be reprogrammed into an "induced pluripotent state (iPS)", and it has recently been shown that reprogramming of human cells is possible [63,64]. However, despite these advances, a number of questions must be answered before iPS cells can be used in human therapies. Although this is an exciting phenomenon, our understanding of the mechanisms involved in reprogramming is still limited.
TISSUE ENGINEERING STRATEGIES FOR BLADDER AUGMENTATION AND REPLACEMENT
As previously noted, the current treatment options for bladder augmentation or replacement are fraught with complications, driving the search for new therapeutic approaches [10,11]. The current standard of care uses gastrointestinal segments for bladder replacement or augmentation. However, the nature and function of gastrointestinal mucosa is quite different than bladder wall tissue, resulting in immediate and long-term complications. The bladder must store urine for prolonged periods and serves as a barrier to prevent highly permeable molecules eliminated in urine from returning to the bloodstream. Specialized cells, called umbrella cells, form the permeability barrier and are located in the apical membrane of the urothelial lining of the bladder lumen. Umbrella cells are interconnected by high resistance tight junctions, which separate the basolateral from the apical cell membranes and block transepithelial ion flux [65,66]. In contrast, gastrointestinal tissues are designed to absorb solutes from the gut lumen into the bloodstream. Therefore, the substitution of gastrointestinal tissue for bladder tissue often leads to metabolic disturbances, urolithiasis, infection, perforation, and increased mucus production. Another complication of prolonged exposure of gastrointestinal tissue to the chemical composition of normal urine is malignancy [10,11,67,68]. To avoid these problems, urologists are participating in Food and Drug Administration human clinical trials to determine the safety and efficacy of regenerative medicine alternatives.
Biomaterial Matrices for Bladder Regeneration
Over the last few decades, several bladder wall substitutes have been attempted with both synthetic and organic materials. Synthetic materials that have been tried in experimental and clinical settings include polyvinyl sponges, Teflon, collagen matrices, Vicryl (PGA) matrices, and silicone. Most of these attempts have failed because of mechanical, structural, functional, or biocompatibility problems. Usually, permanent synthetic materials used for bladder reconstruction succumb to mechanical failure and urinary stone formation, and use of degradable materials leads to fibroblast deposition, scarring, graft contracture, and a reduced reservoir volume over time [33,69].
Because of these issues with synthetic materials, there has been a resurgence in the use of various collagen-based matrices for urological tissue regeneration. Non-seeded allogeneic acellular bladder matrices have served as scaffolds for the ingrowth of host bladder wall components. The matrices are prepared by mechanically and chemically removing all cellular components from bladder tissue [38,70-73]. The matrices serve as vehicles for partial bladder regeneration, and relevant antigenicity is not evident.
Cell-seeded allogeneic acellular bladder matrices have been used for bladder augmentation in dogs [38]. The regenerated bladder tissues contained a normal cellular organization consisting of urothelium and smooth muscle and exhibited a normal compliance. Biomaterials preloaded with cells before their implantation showed better tissue regeneration compared with biomaterials implanted with no cells, in which tissue regeneration depended on ingrowth of the surrounding tissue. The bladders showed a significant increase (100%) in capacity when augmented with scaffolds seeded with cells, compared to scaffolds without cells (30%). The acellular collagen matrices can be enhanced with growth factors to improve bladder regeneration [74].
Small intestinal submucosa (SIS), a biodegradable, acellular, xenogeneic collagen-based tissue-matrix graft, was first described in the 1980's as an acellular matrix for tissue replacement in the vascular field [75]. It has been shown to promote regeneration of a variety of host tissues, including blood vessels and ligaments [76]. The matrix is derived from pig small intestine in which the mucosa is mechanically removed from the inner surface and the serosa and muscular layer are removed from the outer surface. Animal studies have shown that the non-seeded SIS matrix used for bladder augmentation is able to regenerate in vivo [77,78]. Histologically, the transitional layer was the same as that of the native bladder tissue, but, as with other non-seeded collagen matrices used experimentally, the muscle layer was not fully developed. A large amount of collagen was interspersed among a smaller number of muscle bundles. A computer-assisted image analysis demonstrated a decreased muscle-to-collagen ratio with loss of the normal architecture in the SIS-regenerated bladders. In vitro contractility studies performed on the SIS-regenerated dog bladders showed a decrease in maximal contractile response by 50% from those of normal bladder tissues. Expression of muscarinic, purinergic, and alpha-adrenergic receptors and functional cholinergic and purinergic innervation were demonstrated [78]. Cholinergic and purinergic innervation also occurred in rats [79].
Bladder augmentation using laparoscopic techniques was performed on minipigs with porcine bowel acellular tissue matrix, human placental membranes, or porcine SIS. At 12 weeks post-operatively the grafts had contracted to 70%, 65%, and 60% of their original sizes, respectively, and histologically the grafts showed predominantly only mucosal regeneration [80]. The same group evaluated the long-term results of laparoscopic hemicystectomy and bladder replacement with SIS with ureteral reimplantation into the SIS material in minipigs. Histopathology studies after 1 year showed muscle at the graft periphery and center but it consisted of small fused bundles with significant fibrosis. Nerves were present at the graft periphery and center but they were decreased in number. Compared to primary bladder closure after hemi-cystectomy, no advantage in bladder capacity or compliance was documented [81]. More recently, bladder regeneration has been shown to be more reliable when the SIS was derived from the distal ileum [82].
In summary, the multiple studies using various materials as non-seeded grafts for cystoplasty indicate that when biomaterials are used alone, small defects can be repaired, but in the repair of large defects, the urothelial layer was able to regenerate normally, but the muscle layer, although present, was not fully developed [38,70,71,78,83,84]. Studies involving acellular matrices that may provide the necessary environment to promote muscle cell migration, growth, and differentiation are being conducted [18]. With continued research in this area, these matrices may have a clinical role in bladder replacement in the future.
Regenerative Medicine for Bladder Using Cell Transplantation
Regenerative medicine with selective cell transplantation may provide a means to create functional new bladder segments. The success of cell transplantation strategies for bladder reconstruction depends on the ability to use donor tissue efficiently and to provide the right conditions for long-term survival, differentiation, and growth. Various cell sources have been explored for bladder regeneration. Native cells are currently preferable due to their autologous source [34]. It has been shown experimentally that the bladder neck and trigone area has a higher propensity of urothelial progenitor cells [85], and these cells are localized in the basal region [86]. Amniotic fluid and bone marrow-derived stem cells can also be used in an autologous manner and have the potential to differentiate into bladder muscle [60,87] and urothelium [88]. Embryonic stem cells also have the potential to differentiate into bladder tissue [89], although their use is currently banned in many countries for this purpose. Most recently, however, it has been shown that progenitor cells can be derived from voided urine, and these cells are also able to differentiate into urothelial and smooth muscle cells, making them an extremely attractive option for bladder engineering [90,91].
Regardless of their source, it has been shown that human urothelial and muscle cells can be expanded in vitro, seeded onto polymer scaffolds, and allowed to attach and form sheets of cells. The cell-polymer scaffold can then be implanted in vivo. Histologic analysis indicated that viable cells were able to self assemble back into their respective tissue types, and retained their native phenotype [28].
As described above, it has been shown that the urothelium is able to regenerate generously over free grafts, most likely because the urothelium is associated with a high reparative capacity [92]. However, bladder muscle tissue is less likely to regenerate in a normal fashion. As a result of this discovery, it was hypothesized that building a three-dimensional bladder construct in vitro, before implantation, would facilitate the eventual terminal differentiation of the cells after implantation in vivo and would minimize the inflammatory response toward the matrix, thus avoiding graft contracture and shrinkage. The dog study described earlier supports this hypothesis and illustrates a major difference between matrices used with autologous cells (tissue-engineered matrices) and those used without cells [38]. Matrices that were seeded with cells and then used for bladder augmentation retained most of their preimplantation diameter, as opposed to matrices implanted without cells, in which significant graft contraction and shrinkage occurred. In addition, histological analysis demonstrated a marked paucity of muscle cells and a more aggressive inflammatory reaction in the matrices implanted without cells.
The results of these initial studies showed that the creation of artificial bladders may be achieved in vivo; however, it could not be determined whether the functional parameters noted were created by the augmented segment or by the remaining native bladder tissue. To better address this question, an animal model was designed in which subtotal cystectomies followed by replacement with a tissue-engineered organ were performed [41]. Cystectomy-only controls and animals that received bladder replacements made from non-seeded matrices maintained average capacities of 22% and 46% of preoperative values, respectively. However, an average bladder capacity of 95% of the original precystectomy volume was achieved in animals receiving cell-seeded tissue engineered bladder replacements. These findings were confirmed radiographically. The subtotal cystectomy reservoirs that were not reconstructed and the polymer-only reconstructed bladders showed a marked decrease in bladder compliance (10% and 42% total compliance). In contrast, the compliance of the cell-seeded tissue-engineered bladders showed almost no difference from preoperative values that were measured when the native bladder was present (106%). Histologically, the non-seeded bladder replacement scaffolds presented a pattern of normal urothelial cells with a thickened fibrotic submucosa and a thin layer of muscle fibers. The tissue-engineered bladders (scaffold + cells) showed a normal cellular organization, consisting of a trilayer of urothelium, submucosa, and muscle. Immunocytochemical analyses confirmed the muscle and urothelial phenotype. S-100 staining indicated the presence of neural structures [41]. These studies have been repeated by other investigators, and they obtained similar results using larger numbers of animals over the long-term [83,93]. Thus, the strategy of using biodegradable scaffolds seeded with cells can be pursued without concerns for local or systemic toxicity [94].
However, not all scaffold materials perform well if a large portion of the bladder must be replaced. In a study using SIS for subtotal bladder replacement in dogs, both the unseeded and cell seeded experimental groups showed graft shrinkage and poor results [95]. This confirms that the type of scaffold used in the construction of tissue-engineered bladders is critical for the success of these technologies. The use of bioreactors, which provide mechanical stimulation for the growing organ in vitro, has also been proposed as an important parameter for success [96]. Bioreactors provide can provide mechanical stimulation such as periodic stretching of the tissue, which has been shown to assist in in vitro muscle development, and exposure to flow conditions, which is important for the development of endothelial layers in blood vessels and hollow organs such as the bladder. In fact, Farhat and Yeger [96] have developed bioreactor systems specifically for bladder development. These systems provide simulated filling/emptying functions to the engineered tissue, and this may lead to a bladder construct with more functionality.
A clinical experience involving engineered bladder tissue for cystoplasty was conducted starting in 1998. A small pilot study of seven patients reported the use of either collagen scaffolds seeded with cells or a combined PGA-collagen scaffold seeded with cells for bladder replacement. These engineered tissues were implanted with or without omental coverage (Fig. 1). Patients reconstructed with engineered bladder tissue created with cell-seeded PGA-collagen scaffolds and omental coverage showed increased compliance, decreased end-filling pressures, increased capacities and longer dry periods over time (Fig. 2) [97]. This experience suggests that the engineered bladders continue to improve with time, mirroring their continued development. Although the experience is promising and shows that engineered tissues can be implanted safely, it was just a first step towards the goal of engineering fully functional bladders. Two multi-center Phase II clinical studies, the first involving pediatric patients with neurogenic bladder secondary to spina bifida and the second involving adult patients with neurogenic bladder secondary to spinal cord injury, were then undertaken to evaluate the safety and effectiveness of the tissue-engineered Neo-Bladder Augment (NBA). The spina bifida study involved 10 patients (mean age 8.2 years, 6 females/4 males) at 4 medical centers in the United States who required augmentation cystoplasty due to bladder pressures ≥40 cmH2O and/or development of upper urinary tract changes such as hydronephrosis or vesicoureteral reflux. Each patient underwent an open bladder biopsy, and autologous cells derived from the biopsy were expanded in vitro and seeded onto the NBA scaffold. This construct was then implanted into each patient. The procedure was well-tolerated and 6 of the 10 patients showed clinical improvement based on urodynamics studies, radiography, and voiding diary results. The second study involved 6 patients over the age of 18 with severe bladder dysfunction secondary to spinal cord injury. The same procedure was performed to create the implantable construct from the patients' cells and the NBA scaffold, and after 2 years of follow-up, it was found that 4 of the 6 patients responded well to the tissue-engineered bladder. However, both studies highlighted an important issue in bladder regeneration. In both studies, the implants placed patients who were able to undergo normal bladder cycling (filling and emptying) regenerated well, while those implanted in patients who did not have normal bladder cycles due open bladder necks or other physiological issues did not respond as well to this therapy. This further supports the idea that conditioning engineered bladder tissue within a specially designed bioreactor prior to implantation may lead to improved clinical results.

Construction of engineered bladder. (A) Scaffold material seeded with cells for use in bladder repair. (B) The seeded scaffold is anastamosed to native bladder with running 4-0 polyglycolic sutures. (C) Implant covered with fibrin glue and omentum.
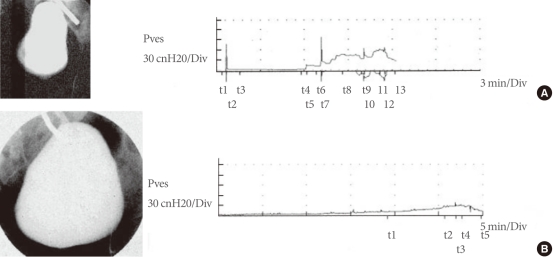
Cystograms and urodynamic studies of a patient before and after implantation of the tissue engineered bladder. (A) Preoperative results indicate an irregular-shaped bladder in the cystogram (left) and abnormal bladder pressures as the bladder is filled during urodynamic studies (right). (B) Postoperatively, findings are significantly improved. Pves, intravesical pressure. (Reprinted from Atala A, Bauer SB, Soker S, Yoo JJ, Retik AB. Lancet 2006;367:1241-6, with permission of Elsevier Limited [97]).
CONCLUSIONS
Regenerative medicine approaches are currently being developed for every type of tissue and organ within the urinary system. Most of the progress within this field has occurred within the last decade. A pipeline of regenerative medicine technologies now exists with products in the discovery stage, preclinical testing, and clinical trials. Research is underway to expand both cell source and biomaterial options for regenerative medicine applications. Recent progress has established the feasibility of using regenerative medicine to treat neurogenic bladder and the future promises that regenerative medical treatment options will expand to patients with other bladder diseases, as well as to patients requiring replacement of other organs throughout the body.
Notes
No potential conflict of interest relevant to this article was reported.